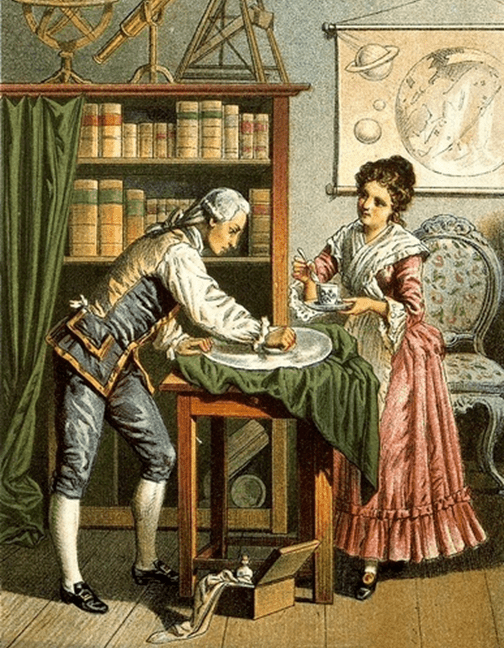
On April 9th, 2021, we had the honor and pleasure of having a member Middy Streeter give a talk. He was gracious enough to allow us to share a recording of his presentation about Jewish Astronomers.
This talk is a survey of some of the major contributions made by Jews to the field of astronomy. Beginning in the 18th century, Middy describes the achievements of Caroline and William Herschel (pictured above) and then highlight further advancements in the field up to the present.
Middy Streeter is an English instructor at Hudson Community College and the author of Solving the Solar Enigma: The Story of the Scientists Behind the Discovery of the Sun’s Energy Source (Dimension Engineering Press). He has also written for Destination: Vietnam; The Circus Report; and The Waterbury Republican-American.
Below the video you can find a longer version of the talk.
Watch a recording of his presentation:
Viewing any subject through the lens of a particular group of people selected strictly because of the circumstances of their birth, is certainly not the best strategy for seeking any kind of deep understanding. But with that said, I hope you’ll find at least some of what I have to say informative regarding a few of the extraordinary astronomers who also happened to be Jewish.
As far as famous astronomers go, Galileo Galilei is still at the top of the heap, even after the four centuries that have passed since his death. And for good reason, his work led to a quantum leap in our knowledge of the heavens. One of the things about his work in that area that I find particularly intriguing is that what he did was something that all of us can imagine ourselves doing: He simply took a piece of available technology and put it to a new use. When the telescope was invented in 1608, he was just another physics professor minding his own business with matters such as developing the concept of relativity that Albert Einstein would later make use of. When he heard about these new–fangled devices being put to military and maritime use, however, he began thinking about what might be learned if one of them were to be trained on the night sky. With that little thought he proceeded to gain even more immortality than he would have with just the Einstein connection.
William Herschel
As far as famous astronomers go, Galileo Galilei is still at the top of the heap, even after the four centuries that have passed since his death. And for good reason, his work led to a quantum leap in our knowledge of the heavens. But that’s all I’m going to say about him because, as we all know, he wasn’t Jewish. And he probably wasn’t all that well known by the general public during his lifetime, except for maybe in the tabloids during his big trial for heresy. The first astronomer to achieve worldwide public acclaim was the British astronomer William Herschel, and he was Jewish. Actually, though, only his father was Jewish, and he converted to Lutheranism. And to add insult to injury, his son John, who also became a notable astronomer, was buried in that bastion of the Anglican Church, Westminster Abbey. Nevertheless, this is not the time to nitpick.
So, when William Herschel began his professional life, it was for his very considerable musical talent that he had the most potential for fame. He was born in 1738 in the Hanover region of what would later become Germany. His father was an oboist with the military band attached to the Hanoverian Guards, and William followed in his footsteps by becoming a musician in that unit as well. When he was nineteen, however, his father sent him to England to avoid the aftermath of the defeat of the Hanoverian Guards in the Battle of Hastenbeck. One advantage that Herschel had was that he was a multi-instrumentalist. In addition to the oboe, he played the violin, harpsichord, and organ. He worked his way up the English musical ladder, doing stints as a violinist with the Newcastle Orchestra and later as the leader of the Durham Militia Band, and in 1766 he was hired as the organist for the Octagon Chapel in the town of Bath.
Significantly, Herschel was also interested in the technical side of music, which led him to read Robert Smith’s book “Harmonics, or the Philosophy of Musical Sounds.” He then decided to read another of Smith’s works, “A Compleat System of Opticks,” and that book happened to have a section on telescopes.
He then decided to build a telescope himself, and the one he chose to make was a reflector telescope. This type of telescope was invented by Isaac Newton and uses a concave mirror instead of a series of lenses like the refracting telescopes that Galileo used. Not satisfied with the quality of the mirrors that were available at that time, Herschel studied lens grinding with a local artisan and constructed a telescope with a seven–inch mirror, the biggest in the country at that time.
It’s not all that clear what came first, his interest in gadgets or his interest in astronomy, but in any event, he certainly put his new toy to good use because he then began to methodically record the position of every celestial object he could find. In 1781, after eight years of doing this laborious work over hundreds of chilly nights in the backyard of his house in Bath, he noticed something moving in an unusual way that he at first thought might be a comet. It turned out to be a planet. The word “planet” goes back to the ancient Greek word for “wanderer.” He wanted to name it “Georgium Sidus,” Latin for “George’s Star,” in honor of King George III, but even though there were probably lots of British astronomers who relished the thought of how annoyed their counterparts in the recently founded United States of America would be at the choice of that name, cooler heads prevailed, and it was eventually named Uranus after the Greek god of the sky. It was the first planet discovered since antiquity, and it made Herschel famous almost overnight. The next year the Royal Society, the most prestigious science organization of that time, awarded him their Copley Medal, which was the Nobel Prize of its day.
It remains a mystery as to why George III didn’t get around to knighting Herschel until 1816, but my suspicion is that it was because he didn’t push harder for the “Georgium Sidus” name. In any event, the only thing I might add is that William Herschel is certainly a lesson to all those parents out there who think their children will never amount to anything if all they do is sit around playing music or tinkering with things.
Caroline Herschel
Herschel was also a really good guy, and the reason is that he helped out his younger sister Caroline when she most needed help. Unfortunately for her, only two of the ten children in the Herschel family were girls, and her sister, sixteen years her senior, married when Caroline was five and old enough to start doing housework. Her mother then began having designs on keeping her as a permanent maid and resisted her efforts to get even a minimal education. Fortunately, in 1772, when she was twenty-two, William rescued her from the clutches of Mama by bringing her to England. Like her brother, Caroline had a remarkable ability to apply herself to whatever task she set for herself. One thing she wanted to do was learn to sing, and soon after arriving in Bath she started taking lessons. In only a couple of years she was singing in professional choral groups.
When William turned to astronomy, Caroline began working closely with him and continued to do so for the rest of their lives together. In the course of all those nippy nights of helping him record his celestial observations, Caroline developed an extensive knowledge of astronomy herself, and whenever she could, she did her own observing. On February 26, 1783, she made her first discovery: a nebula that was not included in any of the star catalogues available at that time. It is now known as Messier 110 and is actually a small galaxy. Three years later on August 1, 1786, she spotted something that resembled a nebula except that it was round, and she correctly identified it as a comet. Comets are loose collections of ice, dust, and small rocky particles that orbit the Sun. One way to imagine them is to think of them as dirty snowballs. Their sizes can vary from a mile to tens of miles in diameter. When they pass close to the Sun, its warmth makes them release gases, and the resulting atmospheres, which often appear as long tails, are visible for relatively brief periods of time.
Caroline went on to discover seven more comets, and five of them were documented in Philosophical Transactions of the Royal Society. She also compiled an update of the Flamsteed star catalogue that contained more than 550 stars that had not been previously included.
In 1787 George III made William his Court Astronomer and hired Caroline as his assistant. Her appointment made her the first woman to work as a professional astronomer. I think we can say that Caroline and William were the closest thing to the Wright brothers that the field of astronomy has ever had.
Vera Rubin
The Herschels’ fame came as a result of their consummate skill in tracking the movements of celestial bodies, and, two hundred years later, Vera Rubin made her name as an astronomer through her ability in that area as well, except that what she was following were galaxies. Her father, born in Vilnius, Lithuania, then part of Poland, changed his name from Pesach Kobchefski to Philip Cooper. Her mother was descended from Jews from the Bessarabian region of Eastern Europe. For her master’s thesis at Cornell in 1951, Rubin studied the rotational motions of 108 galaxies and found similarities that no one before her had detected. She continued doing this research for the next three decades.
While studying the rotation of the Andromeda galaxy, the closest spiral galaxy to our home galaxy the Milky Way, she saw something surprising. When she calculated the velocities of its stars, she discovered that they were all moving at roughly the same speed. She was expecting the stars near the center to be moving more rapidly than the more distant stars. The most familiar example of this situation is our own solar system with the inner planets taking much less time to orbit the Sun than the distant ones. A quick explanation: When a light source is moving away from you the distance between the peaks of the electromagnetic waves reaching you increases, which results in a lowering of their frequency. When this happens, the light’s spectrum appears to be a little redder than usual because light waves on the infrared side of the spectrum have a lower frequency than their ultraviolet counterparts. The same thing happens with sound waves, which is why the pitch of a police car siren gets lower as it moves away from you. The amount of this so–called redshift can thus be used to determine the velocity of the light source – the greater the red shift, the higher the velocity. And speaking of infrared light, it should also be mentioned that it was first detected by William Herschel.
When Rubin spotted this uniformity in the speeds of the stars in the galaxies, she began to consider the possibility that there was a halo of unseen matter exerting a gravitational pull on the outer stars and thereby accelerating their motion. She conservatively estimated that almost half of all the matter in Andromeda was in this form, a form that mysteriously emitted no light whatsoever. Rubin and her colleague W. Kent Ford published their results in 1970.
Over the next ten years she studied some 200 more galaxies and found that the velocities of their stars indicated the presence of invisible matter around every one. She, Ford, and their colleague, Norbert Thonnard, published their results in 1980 in the Astrophysical Journal in a landmark paper with a long title, the first part of which is “Rotational Properties of 21 Sc Galaxies with a Large Range of Luminosities and Radii.” With the release of this paper, the existence of what has come to be known as dark matter was conclusively established. The current estimate is that only 15% of the matter in the Universe is in the form of atoms. The rest is dark matter. Rubin and her team had discovered the most abundant material in the Universe.
Rubin was invited to make many a commencement speech over the course of her long life – she died at the age of 88 in 2016 – and in one of them she said this:
“Each one of you can change the world, for you are made of star stuff, and you are connected to the Universe.”
Not surprisingly, she received many awards for her accomplishments, one of the most prestigious being the Gold Medal of the Royal Astronomical Society. She was only the second female recipient and the first woman to be given it since the year 1828. You can now probably guess that her lone female predecessor was none other than Caroline Herschel.
Saul Perlmutter and Adam Riess
We’re now going to take a quick detour into the work of a gentile astronomer, but not just any such astronomer; this one happens to be the Galileo of the 20th century, Edwin Hubble. In the 1920s he made an extensive study of the velocities at which stars and galaxies were moving away from us, and when he then considered their distances from us, he found a correlation: The more distant stars and galaxies were moving at greater velocities than the nearer ones. He then concluded that the speed of a celestial body could be used to determine its distance from us. Through this single insight he gave us a yardstick to measure the Universe.
There is one type of very distant star, though, whose brightness alone can be used as a distance indicator, and that is a Type Ia supernova. Faint Type Ia supernovas are always farther away than their brighter counterparts. The reason is that this type of supernova only occurs if a star in a binary star system that has aged to the point of becoming a white dwarf merges with its companion star and then achieves a certain critical mass. The burst of light that then occurs has a fairly consistent peak luminosity, which is why the amount of each Type Ia supernova’s brightness can be used to measure its distance from us. Saul Perlmutter and Adam Riess were experts on these kinds of supernovas, and, while observing them in 1998, they and their colleagues noticed something unusual: The more distant ones weren’t as bright as they were expected to be according to the Hubble method of measuring their distances. Their red shifts, in other words, indicated that their velocities, and therefore their distances from us, were not as great as their dimness indicated. Perlmutter, working at the Lawrence Berkeley National Laboratory, and Riess, working separately with Brian Schmidt at Australian National University, then concluded that there had to be more energy pushing these stars outward than was previously thought, a lot more energy, in fact. They calculated that this energy, now known as dark energy, comprises almost three quarters of all of the mass-energy in the Universe. They had thus discovered the most abundant entity in the Universe. All three were awarded the Nobel Prize in Physics in 2011. Just as Vera Rubin had done before them, Saul Perlmutter and Adam Riess dramatically increased our knowledge of the makeup of the Universe.
In addition to their discovery of dark matter, Perlmutter, Riess, and Schmidt’s calculation of just how much of it there is out there led to another mystifying and also paradigm-shifting discovery, and that was that the amount of dark energy is so great that it has created a situation in which the Universe’s rate of expansion is actually increasing.
Just as is the case with dark matter, almost nothing is known about the nature of dark energy. As Adam Riess put it, “We’re really just the frosting on a cake, and we don’t know what’s inside the cake.”
One more thing about Saul Perlmutter, not only is he Jewish, but he was raised as a humanist Jew. Until quite recently, his parents were members of the SHJ congregation in Sarasota, Florida.
Hans Bethe
So far, the astronomers I’ve been talking about were engaged in answering the simple question: “What’s out there?” Now I’m going to talk about astronomers who were trying to answer the question: “What’s going on out there?” And in terms of understanding how astronomical things work, one of the most fundamental questions that needed to be answered was how the Sun and all the other stars were generating their energy. The person who answered that question, Hans Bethe, came to America in as a refugee from Nazi Germany because one of his grandparents was Jewish.
After Albert Einstein developed his E = mc2 equation in 1905, the scientific community became curious as to how matter could have so much energy locked up in it. The “c” in the equation refers to the speed of light, which is 186,000 miles per second, and if you square it and multiply that number by even a tiny amount of matter, you get a huge figure. The answer began to emerge six years later when an experimental physicist at the University of Manchester named Ernest Rutherford discovered that atoms were made up of protons in addition to electrons. As we know, subatomic particles come in three varieties: electrons, protons, and neutrons. The electron was the first to be discovered, and that was in 1897 by the British physicist J. J. Thomson. Rutherford discovered the proton in 1911, and his protégé James Chadwick discovered the neutron two decades later in 1932. One thing about protons and neutrons is that they really like to stick together; so much so, in fact, that the amount of energy keeping them together is on the scale of the speed of light squared, and when protons and neutrons do become separated, an amount of energy on that scale is released. Nuclear power stations make use of this phenomenon, which is known as nuclear fission.
As we also know, things with the same electrical charge repel each other, and so when protons, which are positively charged, are pushed together with enough force to overcome the repulsion of their like charges, a process known as nuclear fusion, even more energy is released than when fission occurs. Not long after Rutherford’s discovery, the British physicist Arthur Eddington began to speculate that stars were using some kind of fusion process to generate their energy. When others in the scientific community scoffed at this idea by saying that stars were not hot enough to create the necessary conditions for nuclear fusion, he memorably retorted, “Then find me a hotter place!”
Unfortunately for Eddington, he was born too soon to be able to solve the mystery of stellar energy generation because neutrons played a key role in the puzzle, and, as mentioned, they weren’t discovered until 1932. But we don’t have to feel too sorry for him, at least as far as fame goes, because he was knighted in 1930.
Hans Bethe had a friend named Edward Teller who was a fellow nuclear physicist and Hitler refugee, and he had a colleague named George Gamow who had also recently escaped from an oppressive regime, in his case Stalinist Russia. Gamow, who would become Vera Rubin’s dissertation advisor at George Washington University a decade and a half later, was organizing a conference in 1938 on the question of stellar energy generation, and Teller invited Bethe to attend. When Charles Critchfield, a student of Teller’s, learned that Bethe would be there, he asked him for assistance in his effort to understand the behavior of protons when they experienced the extremely high heat that occurs in the interiors of stars. Working together, they discovered that under these conditions pairs of protons would collide with each other with enough force that one would emit a positron, thus losing its positive charge and becoming a neutron. The result would then be a hydrogen isotope, consisting of a proton and a neutron, known as a deuteron. This was nuclear fusion at its most elemental level. What they also found, however, was that the creation of deuterons did not release much energy. It was an initial step, though, and Bethe decided to figure out the rest of them.
What he then did was to examine all of the possible nuclear combinations that protons and deuterons might experience under the extreme thermal conditions of the interiors of stars. This work took him two months, but he eventually found what he was looking for: After deuterons were created they combined with protons to form helium atoms, and when that happened his calculations indicated that an amount of energy would be released that was comparable to the energy that stars like the Sun had been observed to emit. He detailed his findings in a paper entitled “Energy Production in Stars” and very belatedly received the Nobel Prize in Physics for this work in 1967.
With the coming of the Second World War, Bethe worked on the Manhattan Project to harness nuclear fission to create an atomic bomb. In 1940, Time magazine called him “one of Nazi Germany’s greatest gifts to the United States.”After the war he chose not to assist Teller and some of his other colleagues in the development of the hydrogen bomb. Instead, he became active in the disarmament movement and worked to educate the public about the dangers of nuclear weapons. He served on the President’s Science Advisory Committee from 1956 to 1959, and in 1958 he made critical contributions to the discussions in Geneva on the banning of nuclear testing. He once wrote,
“No one any longer pays attention to – if I may call it – the spirit of physics, the idea of discovery, the idea of understanding. I think it’s difficult to make clear to the non-physicist the beauty of how it fits together, of how you can build a world picture, and the beauty that the laws of physics are immutable.”
Ralph Alpher
We’ve been talking about people who’ve studied what’s out there, and what’s going on out there, but if we broaden our discussion to include the question “Why is everything out there?”– the question of the origin of the Universe, in other words – we come upon the remarkable contributions of a few more people who also happened to be Jewish.
One person who was particularly intrigued by Bethe’s breakthrough was George Gamow, the nuclear physicist who organized the 1938 conference that proved to be so momentous for him. It was not the fact that Bethe had determined how so much energy was being generated by stars that interested Gamow so much as it was that he had figured out how they were manufacturing a new element, helium, in the process. This breakthrough made him wonder where all of the other elements in the Periodic Table had come from, and his suspicion was that the stars had “cooked” them in some way that might have been similar to the creation of helium. In an effort to find out more, he arranged another conference at GWU in 1942 under the title of “The Problems of Stellar Evolution and Cosmology.”
As it happened, the conference did not provide the answer that Gamow was looking for. Instead, what the discussions pointed to was that any understanding of the origins of the elements would require an understanding of what happened before the creation of the stars. As he put it in the conference report that he co-wrote with John Adam Fleming of the Carnegie Institution’s Department of Terrestrial Magnetism:
“It seems, therefore, more plausible that the elements originated in a process of explosive character, which took place at the ‘beginning of time’ and resulted in the present expansion of the universe.”
The “process of explosive character” that they mentioned later came to be known as the Big Bang, a gigantic burst of energy that created, in an extremely dense form, all the matter that would go on to become the atoms and dark matter that make up the Universe. So, the upshot of the conference was that if Gamow wanted to find out where the elements came from, he was going to have to find out what exactly what was going on at the moment of the Big Bang. And so that’s what he set out to do.
But he knew that he couldn’t accomplish this massive undertaking all by himself, and so whom did he turn to in order to save his bacon? A Jew!
Ralph Alpher, whose Jewish father had immigrated from Belarus, suffered the nightmare that all graduate students live in fear of: after he was well along in the writing of his dissertation at GWU in 1946, a paper was published on precisely the same topic. And it was Gamow who delivered the bad news to him. He entered his office with a copy of the journal in question and said, “Ralph, you’ve been scooped!” He then suggested that he join him in exploring the initial formation of elements. The work they did together would immortalize Alpher and, needless to say, make for a very satisfying silver lining.
Gamow needed the help of someone with highly developed skills in math, and he knew that Alpher was the right man for the job because he’d previously worked as a developer of guided missile systems at the Johns Hopkins University Applied Physics Laboratory. Their goal was to determine the circumstances that made it possible for the creation of helium and, eventually, all of the heavier elements. Gamow’s hypothesis was that the Big Bang produced a hot gaseous soup of neutrons, which decayed into electrons and protons that then combined with other neutrons to become all of the other elements with more than one proton in their nuclei. To validate this idea they needed empirical evidence of the tendency of nuclei to capture neutrons under such extreme temperature conditions, and Alpher found what they were looking for in the experiments being done at that time by Enrico Fermi and his colleagues who were developing the technology for nuclear power generation. He and Alpher were then able to calculate that the temperature at the time of the Big Bang was 109 oK, a figure that was lower than the current estimate of 1032 oK but still well within the correct order of magnitude. They completed their work in 1948 and published their findings in a paper entitled “The Origin of Chemical Elements” in the journal Physical Review. Alpher added further details that year in a subsequent paper in the same publication entitled “A Neutron-Capture Theory of the Formation and Relative Abundance of the Elements.” With these papers, Gamow and Alpher succeeded in developing the first mathematically sound explanation of the beginning of the Universe. Not surprisingly, the current understanding of the Big Bang model of the origin of the Universe differs somewhat from the one Gamow and Alpher described, but it remains consistent with the precepts that they presented in their papers.
Somewhat overshadowing the landmark status of Gamow and Alpher’s paper is the legend that has grown up around it because of Gamow’s decision to arbitrarily include Hans Bethe as one of the authors. He loved a good joke and knew that Bethe, an old friend, would enjoy the fun of being on a list of names that resembled the Greek letters alpha, beta, and gamma. What he didn’t know, though, was that Bethe was on the review committee for submissions to Physical Review and so was among the first to read it. Needless to say, Bethe found the paper to be well worth publishing, and it is now fondly known as the “αβγ” paper. In his book “The Creation of the Universe,” published in 1951, Gamow wrote:
“…and so the name of Dr. Hans A. Bethe was inserted in preparing the manuscript for print. Dr. Bethe, who received a copy of the manuscript, did not object, and as a matter of fact, was quite helpful in subsequent discussions. There was, however, a rumor that later, when the αβγ theory went temporarily on the rocks, Dr. Bethe seriously considered changing his name to Zacharias.”
Alpher continued this work with his colleague Robert Herman, and one of the things they did was to calculate the rate of decline in density of the radiation that was originally emitted by the Big Bang fireball. Their estimate of the current density corresponded to a temperature of approximately 5 oK. In 1949 they wrote a paper entitled “Remarks on the Evolution of the Expanding Universe” that appeared in Physical Review. In it they wrote, “This mean temperature [of 5 oK] for the universe is to be interpreted as the background temperature, which would result from the universal expansion alone.” Their claim that there was actual evidence of the Big Bang in the form of an outer radiation shell of photons whose origins lay in the earliest stages of the Universe was utterly unprecedented, and, as sometimes happens, it was almost immediately forgotten.
Arno Penzias
Mathematical explanations are all well and good, but, at the end of the day, experimental proof is usually needed for the explanation of a phenomenon to be fully accepted. For example, upon its publication in 1915, Einstein’s General Relativity paper was, of course, thoroughly scrutinized by the scientific community; however, it was not until Arthur Eddington used the photographs he took of an eclipse in 1919 to show that the force of the Sun’s gravity could bend the light of the stars behind it that would ordinarily not be visible – something predicted by Einstein’s equations – that the world took full notice of his work. Eddington’s photos were the first tangible evidence validating General Relativity, and they made the front pages of newspapers around the world. In the case of Gamow and Alpher’s scenario for the origin of the Universe, the physical proof came by way of two scientists who were not thinking at all about the Big Bang when they did their groundbreaking research in 1964. Their names were Arno Penzias and Robert Wilson.
Penzias was born in Germany in 1933, and at age six, he was one the Jewish children evacuated to England as part of the Kindertransport rescue operation. Sometime later, his parents fled Nazi Germany for the U.S., and the family settled in New York City in 1940. After receiving his doctorate at Columbia in 1961, he took a job at Bell Labs where he met Wilson when he was hired there two years later.
They decided to work together because they were both interested in learning more about the halo of radiation surrounding the Milky Way that had been recently detected. This phenomenon, however, was far too faint to be seen through standard telescopes. What they needed was an instrument that could detect electromagnetic waves in the radio wave realm of 10 x 10-2 m to 30 x 10-2 m rather than the wavelengths of visible light, which range from 400 x 10-9 m to 700 x 10-9 m. (The wavelengths of the infrared range of radiation that William Herschel discovered vary from 700 x 10-9 m to 1000 x 10-9 m.) Fortunately, Bell Labs had a twenty-foot horn reflector antenna at the Crawford Hill Laboratory site in Holmdel, NJ, that was no longer being used; it suited Penzias and Wilson’s purposes perfectly.
Or so they thought. In order to make sure they were detecting only the radiation from the Milky Way, they had to factor out all of the other sources of radiation that would interfere with the radio waves that they wanted to study. This was quite a laborious task that included eliminating factors such as the heat coming from the reflector antenna itself as well as from the ground underneath it. As they went through the various steps involved in isolating the 21 x 10-2 m wavelength that they estimated was that of the halo, they kept detecting radiation with a 7.3 x 10-2 m wavelength. This wavelength corresponded to a temperature of 7.3 oK, and it was significantly more than the 2.3 oK from the earth’s atmosphere and the 1.5 oK from the ground and the antenna itself. The most notable aspect of this 3.5 oK excess temperature was that it appeared wherever they aimed the antenna.
When Penzias mentioned this unexpected situation to his friend, Bernard Burke, a radio astronomer at M.I.T., Burke recalled having recently seen a preprint of a paper by a Princeton astronomer named James Peebles on the subject of extragalactic radio waves, and he suggested that Penzias take a look at it. Peebles was part of a team led by Robert Dicke that, like Alpher and Herman, had come to the conclusion that there were photons left over from the Big Bang that were emitting a very low level of radiation. That formed a shell
Penzias then called Dicke at Princeton, and he sent him a copy of Peebles’ still-unpublished paper. As soon as they read it, Penzias and Wilson invited Dicke, Peebles, and their colleagues David Wilkinson and Peter Roll, to come to Holmdel. Somewhat to their chagrin, the Princeton team found that the radiation at the 3.5 oK level detected by Penzias and Wilson – now known as the cosmic background radiation – was evidence that there was a shell of low-energy photons around the outer reaches of the Universe. Dicke and his team had predicted this phenomenon and had even made plans to build their own radio telescope to search for the shell. After studying Penzias and Wilson’s findings, Dicke echoed Gamow’s words to Alpher when he remarked to his colleagues, “Boys, we’ve been scooped!”
What Penzias and Wilson had found was not the halo of radiation around the Milky Way but the halo around the Universe.
In the wake of the “αβγ” paper, a number of competing theories of the origin of the Universe sprang up, and, in fact, the Big Bang name was coined as a term of derision by the British astronomer Fred Hoyle, an advocate of the rival “steady state” theory. With the advent of Penzias and Wilson’s discovery, though, all of the other theories rapidly fell away.
As Robert Wilson modestly commented in his acceptance speech for the Nobel Prize in Physics that he and Penzias received in 1978, “We were pleased that the mysterious noise appearing in our antenna had an explanation of any kind, especially one with such significant cosmological implications.”
Carl Sagan
Another Jewish astronomer who certainly deserves to be on the famous list is Carl Sagan. AA Brooklyn native whose father came from Kamianets-Podilskyi, a city in the western part of Ukraine, he specialized in the solar system, and his research was central to the first accurate determination of the temperature of the surface of Venus. He also studied Jupiter’s clouds, the seasonal changes on Mars, and the production of amino acids from basic chemicals by radiation to gain a better understanding of the possibility of extraterrestrial life.
Sagan is best known, of course, for having made astronomy more accessible to the general public through his insightful writings and compelling television documentaries. And he also has a connection to Humanistic Judaism in that his daughter Sasha was featured in the Fall 2019 issue of Humanistic Judaism Magazine in connection with a book she wrote about being Jewish in the 21st Century entitled “For Creatures Such as We.”
Sagan’s widow and literary collaborator, Ann Druyan said this about him in a recent interview with Scientific American magazine: “One of the things that I always think is that there are more people who love Carl now than at any previous time, especially because we find ourselves in such a tragic, dark, frightening moment in history, and I think more people are hungry for someone with that kind of voice, a voice that’s not self-serving, that is about the long-tem future, is about the reality of nature… someone who is speaking to communicate, to awaken.”
The observatory Vera Rubin and her colleagues are working in is the Lowell Observatory in Flagstaff, AZ, where she did some of her most important research in the course of her discovery of dark matter. This observatory has been operating since 1894 when it was built by Percival Lowell. He was particularly interested in the planet Mars, and through his extensive observations he became a leading authority on it. He lectured widely, and, beginning in 1895, he also wrote several books for the general public, some of them becoming bestsellers.
In any event, through his work Lowell brought about a new wave of interest in astronomy at the beginning of the 20th century. He was also my great grandmother’s older brother, and although Uncle Percy, as we descendants fondly call him, did not happen to be Jewish, I am extremely proud to say that he was the Carl Sagan of his day.
Needless to say, there are many more Jews who did highly noteworthy work in astronomy, but I hope this short list can at least serve as a good starting point for anyone interested in this subject.
To see upcoming events or watch them live, check out our calendar